Bodyguards against the cold: Ice-binding proteins in Plants
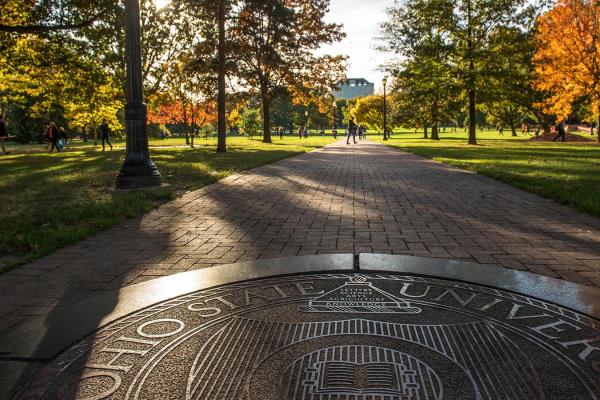
You’ve probably heard many a story of despaired farmers over lost citrus crops or home gardeners over blackened flowers and ornamental plants – all caused by frost and freezing temperatures. Yet, not all plants succumb to the cold. Look out any window and you’ll likely catch a glimpse of something green even on a treacherously cold day.
The simple, captain-obvious reason for this sight is because plants are either freeze-tolerant or freeze-sensitive. Scientists have researched this phenomena and discovered a type of protein that tackles ice crystals growing inside plant leaves and ultimately prevent their growth into tissue-destructing sizes.
Called ice-binding proteins, or IBPs for short, these proteins occupy the inside space of a leaf, or the apoplast. This air-filled region of leaves provides room for gas-exchange, water transport, and sugar transport during photosynthesis. The apoplast is therefore an easy place for water to crystalize into ice once temperatures drop far enough. As this apoplastic water freezes it also expands, creating jagged edges that could puncture cells to the point of no return.
Freeze-tolerant plants have been extensively studied in efforts to understand why they are not damaged by cold. A recent review article published in Frontiers in Plant Science by Bredow and Walker nicely examines the role of IBPs during ice formation in plants. IBPs are conserved proteins found not only in plants, but also cold-tolerant fish and microbes. One way IBPs increase freezing tolerance of plants is to simply lower the freezing point of the apoplast liquid. The paper notes, however, that “In some cases, IBPs have secondary functions not obviously associated with freezing point depression including PR activities, transcriptional regulation, membrane stabilization, ice adhesion, and ice structuring.”
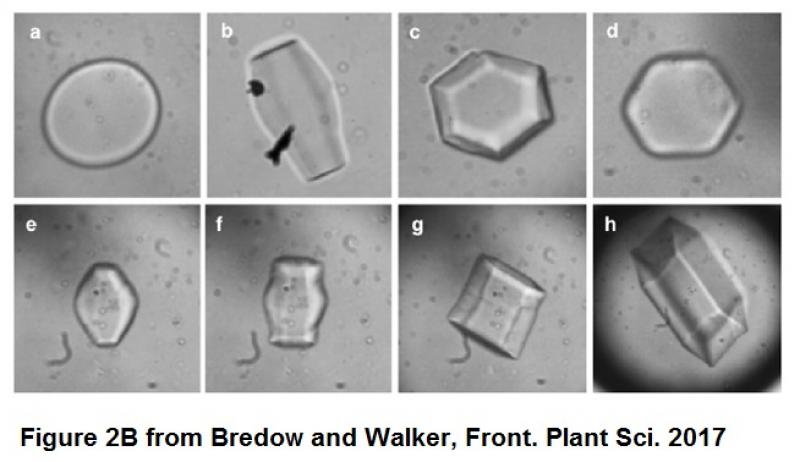
The ice-structuring function of IBPs is rather interesting. Bredow and Walker explain that ice crystals formed from water or buffer have different shapes than crystals containing the ice-binding proteins. In short, when observing morphologies of ice crystals under a microscope, plain water freezes into a disk-like shape, whereas water plus the IBPs freezes into hexagonal crystals (see Figure 2B). Ultimately, the ice-IBP interaction is irreversible, therefore inhibiting further growth of the ice crystal. The authors continue “With the ice crystal unable to grow further at this temperature, the liquid surrounding the ice remains in a supercooled state, resulting in a depression of the freezing point.”
While the impact IBPs could have on food crops and the ornamental plant industry is huge, a lot more study needs to be done. Bredow and Walker lament that only 15 IBPs isolated from plants have been sequenced, and only one of those proteins has had its crystal structure determined. Transgenic studies using IBPs from fish and insects did not produce impressive increases in freeze-tolerance. While little has been done using plant-derived IBPs, these studies showed much more promise as “In all cases the transgenic plants demonstrated decreased electrolyte leakage, indicating membrane protection, and enhanced freeze survival at temperatures below –5C, depending on the sequence and the host.”
So, perhaps with a little more time, we’ll begin seeing freeze-tolerant crops on the market, which would not only help the economy, but also increase global food production.
Written by TPS Fellow, Irene Gentzel
Citation:
Bredow M and Walker VK (2017) Ice-Binding Proteins in Plants. Front. Plant Sci. 8:2153. doi: 10.3389/fpls.2017.02153